If you’ve ever had the chance to explore an ore deposit, or if you’ve even had the chance to look at mineralisation in drill core, or a hand sample, there’s a good chance you’ve thought about this question. We all know the formation of ore deposits are generally associated with a regional-scale tectonic/thermal/magmatic event, which can often form over millions to tens of millions of years. However, the formation of an ore deposit, how long does it take? In this week’s blog we will cover a few examples of ore deposits where this question has been assessed.
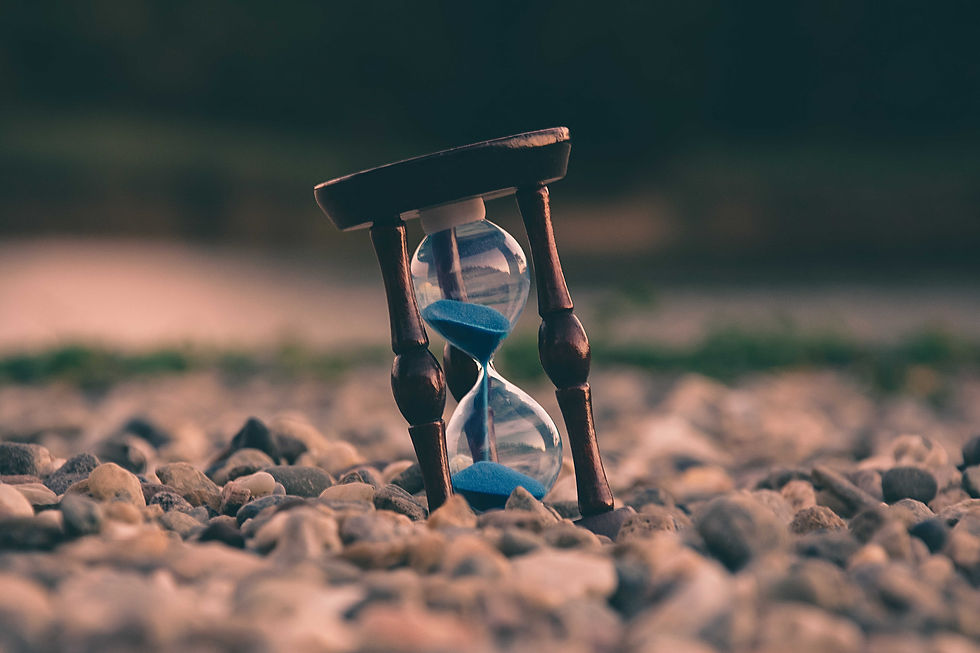
Regolith/Sedimentary Ore Deposits
If we steer away from hydrothermal deposits for a second and consider non-hydrothermal ore deposits such as heavy mineral sands or alluvial gold, deposition occurs primarily through sedimentary processes, which can have a huge range in deposition time. Ultimately, for these deposits, the deposition is a function of the sedimentary environment and relies on a variety of factors that will increase/diminish the effect of physical and/or chemical weathering. Other deposits, such as the Zudong regolith-hosted HREE deposit in China formed through the weathering of underlying HREE-rich granites (Li et al., 2019) (Also - if you're interested further in this topic, click here). Ultimately, it is difficult to assign an absolute number to the time it takes for these deposits to form and likely ranges from decades to centuries to millions of years.

Sedimentary Exhalative (SEDEX) Deposits
The typical SEDEX model involves the formation of stratabound ore through the ascent of moderately hot (>150°C), metal-rich hypersaline basinal brines through active deep-seated faults into the water column (Emsbo, 2009). Although there are many variations on this deposit type, including the diagenetic model, which has been proposed for the Teena and McArthur River Zn-Pb-Ag deposits (Magnall et al., 2021; Spinks et al., 2021), for the purpose of this article, we will only discuss the “classic” SEDEX model. Because of the simplicity of the SEDEX model, the time of deposition must be constrained to the age of deposition of the host rocks. Estimates for individual mineralisation events range from 75,000 to >1 million years (Emsbo, 2009). There are two ways to estimate the time of ore deposit, high-precision stratigraphy (or biostratigraphy) and accompanying geochronology, or modelling the evolution of the deposit. The first case study in this article looks at the Driftpile Ba-Zn-Pb deposit, Northern British Columbia (Nelson et al., n.d.). This deposit formed within the lower to middle Palmatolepis marginifera conodont zone (a defined zone constrained from biostratigraphy), indicating that this deposit formed within a period less than 500 kys (Kaufmann, 2006). With regards to the modelling the metallogenic process, a recent paper by Sheldon et al (2021) indicated that it would take roughly 0.4-0.9 Myr to deposit 20 Mt of Zn-Pb-Ag ore using the classic SEDEX model at the McArthur River Zn-Pb-Ag deposit. If the diagenetic model is invoked, the mineralization process is suggested to be significantly slower, taking ~2.5 Ma to deposit the same amount of ore. However, physical factors that can affect this estimate include heat flux, metal concentration, host rock characteristics and fluid flow rate.
Magmatic-Hydrothermal Ore Deposits
When ore deposits are formed through the infiltration of fluids into a pre-existing rock, the timing and duration of mineralisation can only be determined by performing high precision geochronology on rock units or minerals that have robust petrological constraints with respect to mineralisation. Because of this, we have clumped Skarn, Porphyry and IOCG deposits into a single category and will discuss examples from all three deposit types.
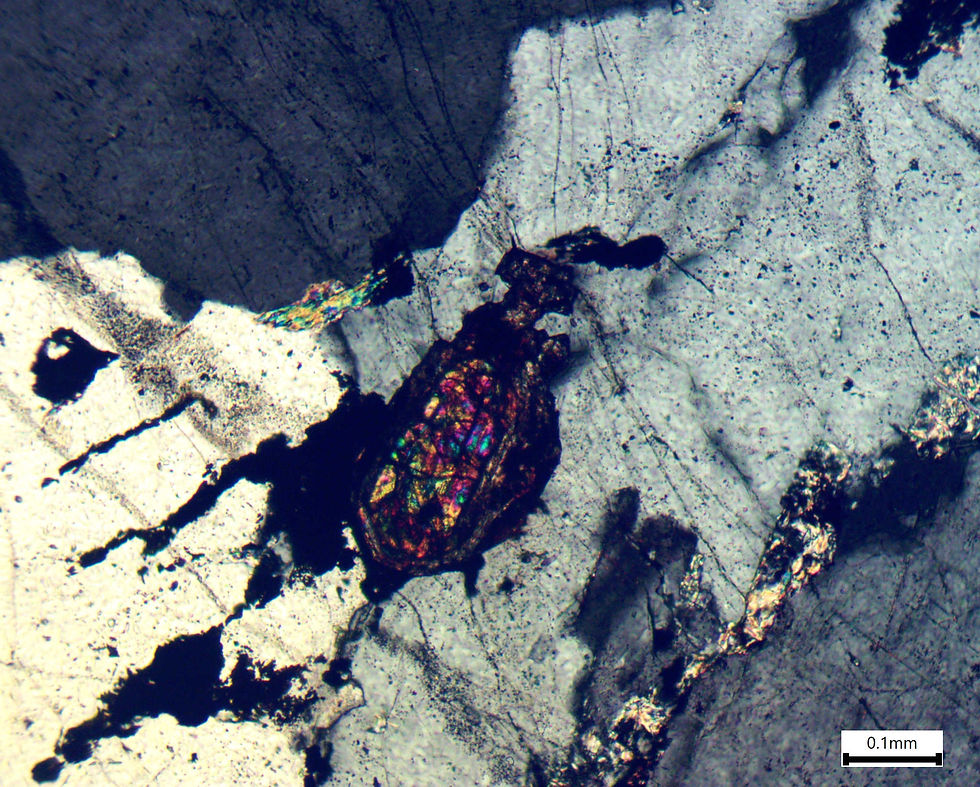
Cu-Au Porphyry: A few studies have used high precision geochronology to understand the time scale of porphyry ore deposits. Large et al (2020) used high-precision geochronology on zircons from various tonalite units to bracket the absolute age of mineralisation at the Batu Hijau porphyry Cu-Au deposit. The youngest zircon from the pre- to syn-ore tonalite records a minimum age of 3.697 ± 0.018 Ma, while the oldest tonalite, which crosscuts Cu-Au mineralisation produces an age of 3.646 ± 0.022 Ma (Large et al., 2020). Based on the difference between these ages, it was concluded that mineralisation occurred over a maximum period of 122 kys (Large et al., 2020). The same method had been applied earlier by a study focused on the age and duration of mineralisation at the Bingham Canyon and Bajo de la Alumbrera porphyry deposits (Von Quadt et al., 2011). In this study, high precision U-Pb geochronology was performed on zircons from pre-ore and post-ore intrusions. It was shown that the age gap between pre-ore and post-ore intrusions ranged from 0.32 Ma and 0.09 Ma from the Bingham Canyon and Bajo de la Alumbrera porphyry deposits, respectively (Von Quadt et al., 2011). This study concluded that single episodes of Cu-Au mineralisation likely occur on a time scale of <100 kys, and possibly much faster (Von Quadt et al., 2011).
Mo Skarn: High-precision geochronology has been performed on the host rocks and corresponding ore minerals at the Xiaojiayingzi Mo Skarn deposit, North China Craton (Ouyang et al., 2023). The Xiaojiayingzi Mo Skarn deposit is sits adjacent an intrusion complex composed of a gabbroic diorite, a monzodiorite, and a granite porphyry unit (Ouyang et al., 2023). This study used high precision zircon U-Pb geochronology, and high precision Re-Os molybdenite geochronology in order to assess the temporal relationship between various intrusive units and Mo mineralisation. This study showed that the intrusion complex formed over a period of ~250 kys, from 165.359 ± 0.18 Ma to 165.099 ± 0.18 Ma (Ouyang et al., 2023). This is synchronous with the formation of exoskarn Mo mineralisation, which was deposited over a period of 450 ± 40 k.y. from 65.48 ± 0.85 Ma to 165.03 ± 0.85 Ma (Ouyang et al., 2023). A subsequent stage of quartz-Mo veins post-date the age of the intrusive complex and formed at 163.73 ± 0.86 and 163.11 ± 0.86 Ma (Ouyang et al., 2023). Unlike the porphyry deposits, which constrain the mineralisation process to <100 kys, it is suggested that Mo mineralisation in the Xiaojiayingzi Mo Skarn deposit was deposited during two discrete episodic pulses over a period of 2.4 Ma (Ouyang et al., 2023).
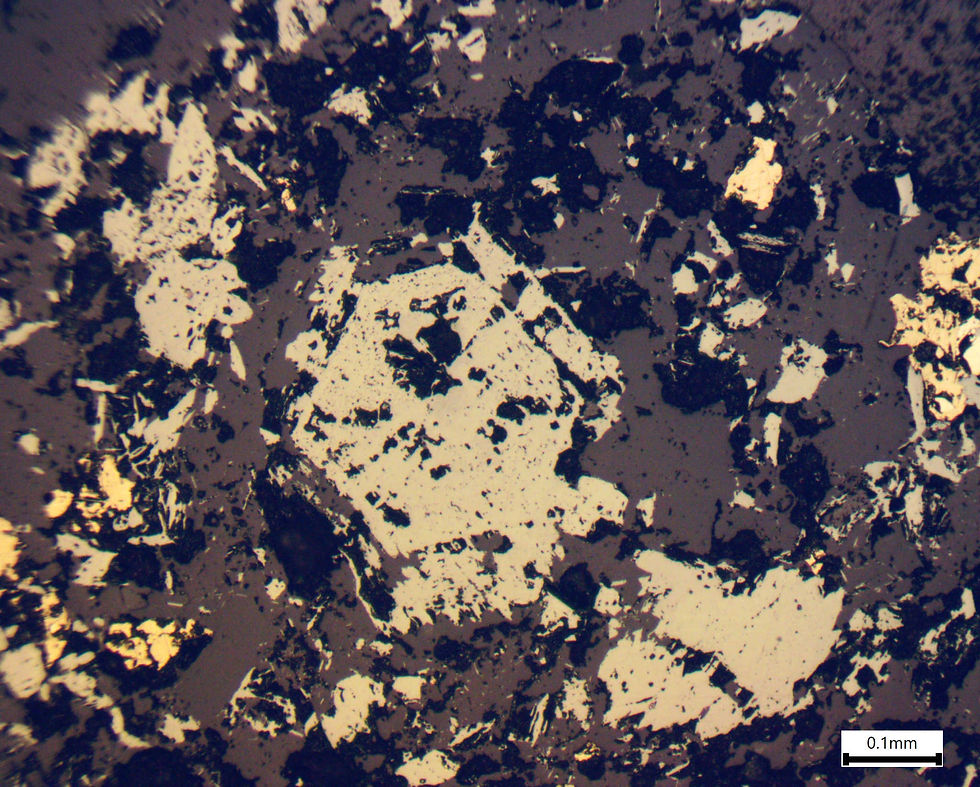
Iron-Oxide-Copper-Gold (IOCG): The Olympic Dam IOCG deposit is one of the largest Cu deposits in the world with a Cu resource of >9500 Mt of ore at 0.82% Cu (Ehrig et al., 2012). Although somewhat off topic for this blog post, the study mentioned here does not resolve the timescale of mineralisation, but the gap between the crystallisation of the host granite, cupola collapse and the formation of IOCG mineralisation. This study used high-precision U-Pb zircon geochronology to constrain the host rock crystallisation age to 1593.28 ± 0.26 Ma and used high-precision hematite U-Pb geochronology to constrain the maximum age of IOCG mineralisation to 1591.27 ± 0.89 Ma (Courtney-Davies et al., 2020). This ~2 Ma time gap is interpreted to represent a magmatic-hydrothermal window in which the crystallisation of the granite was followed by a period of uplift and cupola collapse then IOCG mineralisation (Courtney-Davies et al., 2020). Although this study doesn’t bracket the duration of mineralisation, it serves as an interesting example demonstrating that giant IOCG deposits may form over millions of years.
Conclusion
Like all things geology and science, the question that this article aims to answer is complicated. As mentioned above, the ultimate answer is that it depends on the deposit type. Regolith-hosted and sedimentary deposits may form in a matter of centuries or thousands of years, while SEDEX deposits may form within a period of <1 Ma. Magmatic-hydrothermal deposits show a fairly wide variation, with various porphyry deposits forming within <100 kys, while the giant Olympic Dam IOCG deposit, and the Xiaojiayingzi Mo Skarn deposit likely formed over a period >2 Ma.
References
Courtney-Davies, L., Ciobanu, C.L., Tapster, S.R., Cook, N.J., Ehrig, K., Crowley, J.L., Verdugo-Ihl, M.R., Wade, B.P., Condon, D.J., 2020. Opening the magmatic-hydrothermal window: high-precision U-Pb geochronology of the Mesoproterozoic Olympic dam Cu-U-Au-Ag deposit, South Australia. Econ. Geol. 115, 1855–1870.
Ehrig, K., McPhie, J., Kamenetsky, V., 2012. Geology and mineralogical zonation of the Olympic Dam iron oxide Cu-U-Au-Ag deposit, South Australia.
Emsbo, P., 2009. Geologic criteria for the assessment of sedimentary exhalative (sedex) Zn-Pb-Ag deposits. US Geol. Surv. open-file Rep. 1209, 21.
Kaufmann, B., 2006. Calibrating the Devonian Time Scale: a synthesis of U–Pb ID–TIMS ages and conodont stratigraphy. Earth-Science Rev. 76, 175–190.
Large, S.J.E., Wotzlaw, J.-F., Guillong, M., von Quadt, A., Heinrich, C.A., 2020. Resolving the timescales of magmatic and hydrothermal processes associated with porphyry deposit formation using zircon U–Pb petrochronology. Geochronology 2, 209–230.
Li, M.Y.H., Zhou, M.-F., Williams-Jones, A.E., 2019. The genesis of regolith-hosted heavy rare earth element deposits: Insights from the world-class Zudong deposit in Jiangxi Province, South China. Econ. Geol. 114, 541–568.
Magnall, J.M., Hayward, N., Gleeson, S.A., Schleicher, A., Dalrymple, I., King, R., Mahlstadt, N., 2021. The Teena Zn-Pb deposit (McArthur basin, Australia). Part II: carbonate replacement sulfide mineralization during burial diagenesis—implications for mineral exploration. Econ. Geol. 116, 1769–1801.
Nelson, J., Paradis, S., Farmer, R., n.d. GEOLOGY OF THE DRIFTPILE STRATIFORM, SEDIMENT-HOSTED Ba.-Zn-Pb DEPOSIT, NORTHERN BRITISH COLUMBIA (94W4).
Ouyang, H., Gaynor, S.P., Selby, D., Mao, J., Shu, Q., Li, C., 2023. High-Precision Geochronology of the Xiaojiayingzi Mo Skarn Deposit: Implications for Prolonged and Episodic Hydrothermal Pulses. Econ. Geol. 118, 485–507.
Sheldon, H.A., Schaubs, P.M., Blaikie, T.N., Kunzmann, M., Poulet, T., Spinks, S.C., 2021. 3D thermal convection in the Proterozoic McArthur River Zn-Pb-Ag mineral system, northern Australia. Ore Geol. Rev. 133, 104093.
Spinks, S.C., Pearce, M.A., Liu, W., Kunzmann, M., Ryan, C.G., Moorhead, G.F., Kirkham, R., Blaikie, T., Sheldon, H.A., Schaubs, P.M., 2021. Carbonate replacement as the principal ore formation process in the Proterozoic McArthur River (HYC) sediment-hosted Zn-Pb deposit, Australia. Econ. Geol. 116, 693–718.
Von Quadt, A., Erni, M., Martinek, K., Moll, M., Peytcheva, I., Heinrich, C.A., 2011. Zircon crystallization and the lifetimes of ore-forming magmatic-hydrothermal systems. Geology 39, 731–734.